The Solar/Supercritical CO2 Thermal and Energy Enhancement Laboratory (STEEL) was developed to further research in advanced heat exchangers for a variety of applications such as solar power generation, thermal desalination, waste heat utilization, and solar fuels.
The STEEL facility is equipped with a 7-m parabolic solar dish that is capable of concentrating sunlight by nearly 1000 times at the focal area. The facility is also home to a high pressure (200 bar), high temperature (up to 700 C) supercritical carbon dioxide (sCO2) flow loop. The combination of the parabolic dish and the sCO2 loop give the UC Davis STEEL lab a unique set of capabilities in the area of high temperature and high pressure heat transfer research, along with heat exchanger technology development.
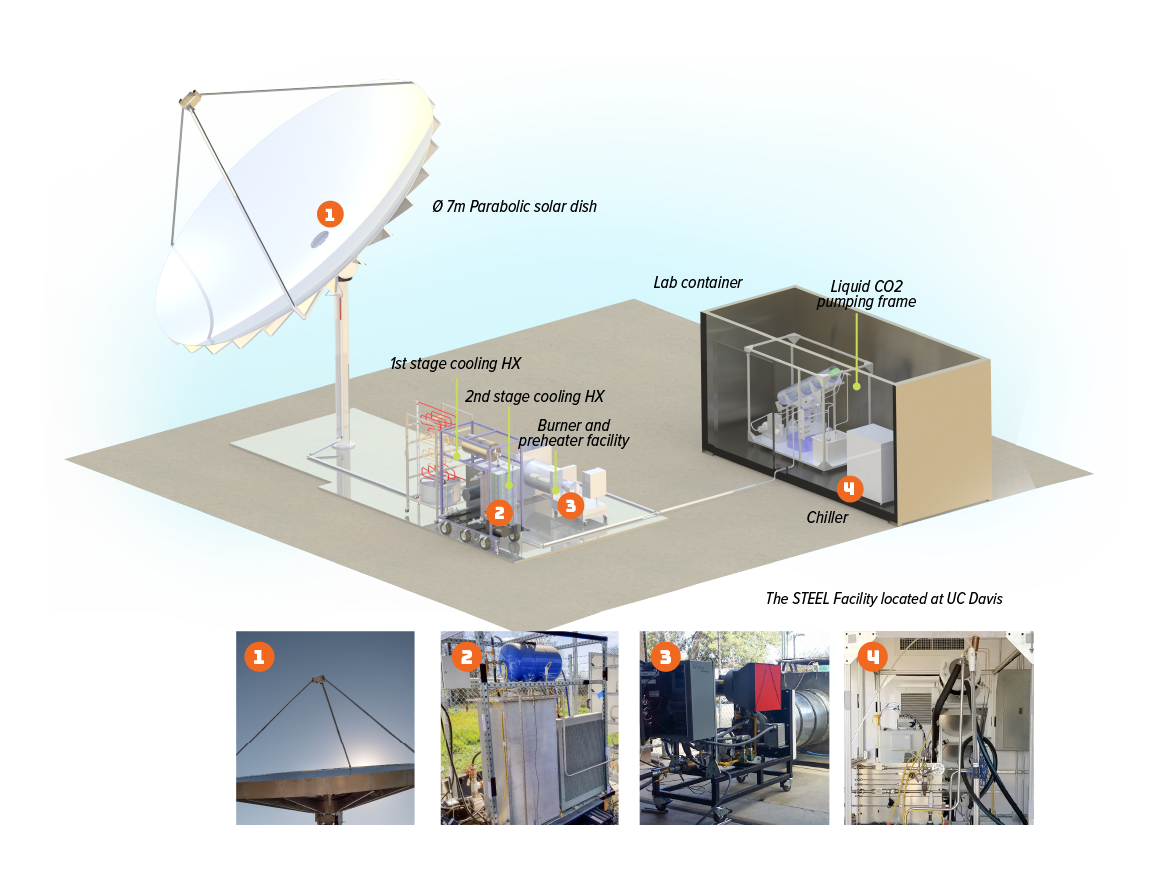
Metal addictively manufactured high T&P microchannel heat exchangers
High efficiency power cycles need to operate at higher turbine inlet temperatures. One such promising cycle, the supercritical carbon dioxide cycle, operates at pressures in excess of 250 bar and temperatures as high as 700-1000 °C at the turbine inlet. Heat Exchangers (HX) account for 40-50% of the total cost of the power cycle and have to withstand thermal stress, creep and fatigue, and in some cases work in a corrosive environment. There is no HX in the market that can meet the needed temperature, pressure, lifetime and corrosion targets. Traditional manufacturing methods impose several constraints on the design of compact heat exchangers for this application. To solve these challenges, an inter-disciplinary team of researchers from UC Davis WCEC and Carnegie Mellon University (CMU) are investigating novel HX designs using metal Additive Manufacturing (AM).
We aim to design a compact HX while minimizing cost, since the materials and methods used for manufacturing are expensive. Compact designs require co-design of thermal-fluidic and mechanical aspects along with manufacturability using metal AM. Concurrent process-based cost modeling by CMU based on our thermo-fluidic models will allow for HX cost optimization.
We developed correlation-based models to predict the HX performance and carried out a parametric study to find out the best geometry dimensions based on the desired HX thermal load. The HX designs have been studied thermally and mechanically through a series of Computational Fluid Dynamic simulations and Thermal-Mechanical structural analysis to ensure high efficiency and reliable designs. Several AM test parts are being made by our partner at CMU and feedback is being incorporated into the HX designs to enable successful fabrication.
Subscale and eventually full scale HXs will be fabricated by CMU and will be experimentally characterized at UC Davis.
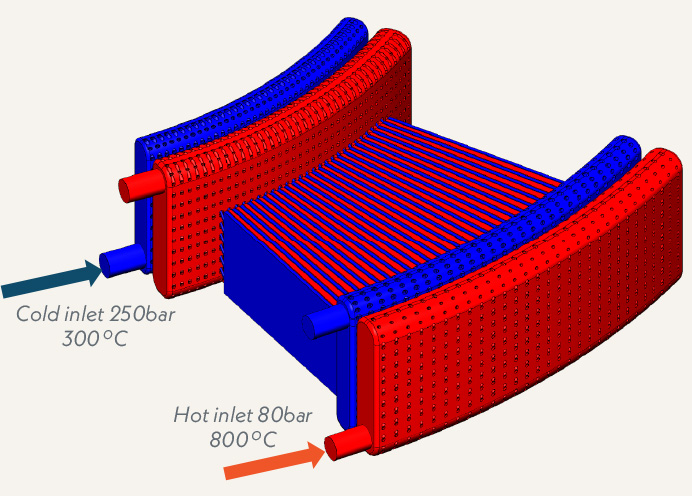
Conceptual design of fluidic bodies within the HX that illustrates the placement of hot (red) and cold (blue) streams next to each other
Microchannel polymer heat exchangers
The path to electrifying heating in California will require new advances in heat pump technology. The new technologies must achieve high efficiency in order to match the operating cost of furnaces that use relatively low-cost natural gas. In addition, new technologies must also consider future regulations on the global warming potential of refrigerants, which is expected to be limited to <750 by the California Air Resources Board in the next few years. In order for new heat pump technologies to really make an impact on the California market, the cost of heat pumps must also come down relative to competing high efficiency heat pumps on the market today. Air-to-water heat pumps suffer from an inherent efficiency penalty when using a secondary loop for delivering the heating and cooling to the home. Improving the efficiency through advanced water-to-air heat exchangers made from cheaper materials will have a significant impact on the potential for these systems moving forward. As a potential solution, our experimentally validated lab-scale microchannel polymer heat exchangers (MPHX) technology has been funded by California Energy Commission to be scaled up for heat exchange thermal load up to 2 Ton (7 kW). We are exploring available commercial fabrication techniques in conjunction with design modifications to enable MPHX technology scale up while keeping the cost feasible.
PREVIOUS WORK
2019
HVAC refrigerants with low global warming potential have significant environmental benefits, but also have flammability concerns; in the interest of safety they are best kept in hermetically, factory-sealed heat pump packages outside homes. To do this, a secondary fluid, such as water, is needed to transfer heat between the air within the HVAC air handler and the refrigerant outside the home. The configuration of a water-to-air heat exchanger (HX) is critically important in determining its effectiveness in terms of thermal performance. Typical finned-tube HXs in air handlers and current state-of-the-art, chilled, water-to-air HXs have significant limitations.
This project, funded by the U.S. Office of Naval Research, uses advances in microchannel technology and additive manufacturing (AM) of plastics to develop a low-cost, highly-effective Microchannel Plastic Heat Exchanger (MPHX). This MPHX could improve conventional air handlers, saving energy and reducing cost.
Based on our prior experience in heat recovery applications, we proposed a conceptual design for a MPHX that consists of multiple “water plates” (WP) in parallel through which cooled or heated water flows. The WPs are joined together by inlet and outlet headers. The internal architecture of each WP consists of a staggered array of microscale pins around which water flows. The WPs are attached together laterally by external fins that form honeycomb air flow passages. Air flows between the WPs and through the fins and exchanges heat with water within the plates.
Results
We developed a thermo-fluidic model for performance predictions of this concept. The performance of MPHX is compared with a finned tube HX. The calculations show that for identical approach temperatures and flow rates the effectiveness of MPHX increased 35% compared to the finned tube design.
RESOURCES
NEWS
- Vinod Narayanan Selected for $4.6M Department of Energy Grant
- DOE Project File: Oregon State University
- DOE Sunshot Program: High Flux Solar Receiver
- DOE Project Profile: University of California, Davis
- ARPA-E High Energy Density Modular Heat Exchangers through Design, Materials Processing, and Manufacturing Innovations
- UC Davis Selected to Receive $2.2 Million Award to Advance Solar Technologies
- Rollett receives $2.4M from ARPA-E for 3D printing research
- Designing the Most Effective Heat Exchanger
PUBLICATIONS
- High Efficiency Microchannel Polymer Heat Exchangers for Heating and Cooling Applications, 2020
- Design and Performance Characterization of a Micro-pin fin sCO2 Recuperator, 2018
- Design and Performance Characterization of an Additively Manufactured Primary Heat Exchanger for sCO2 Waste Heat Recovery Cycles, 2018
Sponsor: DOE Solar Energy Technologies Office; DOE ARPA-E HITEMMP Program
Collaborators: Carnegie Mellon University; National Renewable Energy Laboratory; National Energy Technology Laboratory; HEXCES (division of Clean Energy Systems); GE Global Research; Metal Powder Works LLC; Carpenter Technology Corporation; Special Metals Corporation